Epidemiology and molecular characterization of Enterobacteriaceae producing extended-spectrum β-lactamase in intensive and extensive breeding animals in Burkina Faso
Soufiane Sanou, Adoul Salam Ouedraogo, Manon Lounnas, Arnaud Zougmore, Armel Pooda, Jacques Zoungrana, Georges Anicet Ouedraogo, Rasmata Traore-Ouedraogo, Oumar Ouchar, Hélène Jean-Pierre, Sylvain Godreuil
Corresponding author: Soufiane Sanou, Bacteriology Laboratory Centre Muraz, Muraz Center, Mamadou Konate Avenue, 01 BP 390 Bobo Dioulasso 01, Burkina Faso
Received: 31 Jan 2022 - Accepted: 15 Apr 2022 - Published: 20 May 2022
Domain: Bacteriology
Keywords: Extended-spectrum β-lactamases, animals, livestock, Burkina Faso, antibiotic resistance
©Soufiane Sanou et al. PAMJ - One Health (ISSN: 2707-2800). This is an Open Access article distributed under the terms of the Creative Commons Attribution International 4.0 License (https://creativecommons.org/licenses/by/4.0/), which permits unrestricted use, distribution, and reproduction in any medium, provided the original work is properly cited.
Cite this article: Soufiane Sanou et al. Epidemiology and molecular characterization of Enterobacteriaceae producing extended-spectrum β-lactamase in intensive and extensive breeding animals in Burkina Faso. PAMJ - One Health. 2022;8:4. [doi: 10.11604/pamj-oh.2022.8.4.33553]
Available online at: https://www.one-health.panafrican-med-journal.com/content/article/8/4/full
Research 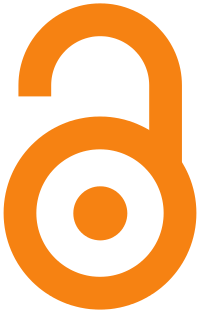
Epidemiology and molecular characterization of Enterobacteriaceae producing extended-spectrum β-lactamase in intensive and extensive breeding animals in Burkina Faso
Epidemiology and molecular characterization of Enterobacteriaceae producing extended-spectrum β-lactamase in intensive and extensive breeding animals in Burkina Faso
Soufiane Sanou1,&, Adoul Salam Ouedraogo2,
Manon Lounnas3,4,
Arnaud Zougmore2, Armel Pooda5,
Jacques Zoungrana5, Georges Anicet Ouedraogo6, Rasmata Traore-Ouedraogo7, Oumar Ouchar3,4, Hélène Jean-Pierre3, Sylvain Godreuil3,4
&Corresponding author
Introduction: extended-spectrum β-lactamases (ESBL) determinants have been detected in clinical isolates and commensal bacteria from humans and animals. We investigated, the ESBL-producing Enterobacteriaceae in stool from intensive and extensive breeding animals (cattle, pigs, and poultry) in Burkina Faso.
Methods: we identified our bacterial strains by MALDI-TOF. Antimicrobial susceptibility was tested with Kirby Bauer method and ESBL genes identified by conventional Polymerase Chain Reaction (PCR).
Results: from March to June 2017 in the Bobo Dioulasso area, we investigated stool samples collected from healthy animals (cattle = 251; pigs = 250 and poultry = 397) in one (1) slaughterhouse, five (5) livestock farms, and one (1) poultry market. The frequency of ESBL gene carriage was 41.03% among cattle, 69.60% among pigs, 0.8% among intensive farming, and 19.1% among extensive poultry farming. Only all the poultry were fed with antibiotics. The bacterial strains carrying the ESBL were E. coli (278/315) and K. pneumoniae (36/315). The ESBL genes carried were CTX-M 15, TEM, and Oxa-1-like. These three β-lactamase genes were associated in some bacterial strains. The E. coli strains belonged most commonly to the phylogroup A.
Conclusion: this high level of resistance of Enterobacteriaceae to antibiotics in livestock in Burkina Faso by the production of ESBL, could suggest environmental contamination of the livestock with ESBL-producing bacteria.
Antimicrobial resistance (AMR) is a global public health threat. The first global surveillance report on antibiotic resistance (ABR) published by WHO in 2014, shows that 45% of deaths in both Africa and South-East Asia were due to multi-drug resistant (MDR) bacteria [1]. Antimicrobial use, misuse, or overuse in clinical medicine is a major contributing factor in the development of AMR in human populations [2-4]. As in clinical medicine, antimicrobials are also widely used in domestic animals and livestock [5]. Sub-therapeutic doses of antimicrobials are used for growth promotion in some countries [6]. This antimicrobial exposure is thought to be an important selective pressure for AMR in animals [7]. Conversely, studies have also shown that various multidrug-resistant bacteria lineages from animals also appear in humans [8,9]. Generally, the AMR bacteria colonize the gut of animals and might play an epidemiological role in the spread of resistance between Foods Producing Animals (FPA) and humans, either through direct contact or consumption of contaminated meat [10].
According to the Food and Agriculture Organization of the United Nations (FAO), worldwide, pork (36.3%) chicken (35.2%), and beef (22.2%) are the most common meat sources [11]. Production of extended-spectrum β-lactamases (ESBLs) is the most common mechanism of resistance to third-generation cephalosporins among Enterobacteriaceae [12,13]. These resistant strains are considered a significant public health issue due to the limited therapeutic [1] options and increased morbidity and mortality associated with them [2]. The true prevalence of ESBL is not well-known in Africa and is probably underestimated because of the paucity of studies in human health, animal health, and the food chain on the continent [14]. Here, we investigate the epidemiology and molecular characterization of Enterobacteriaceae producing extended-spectrum β-lactamase in intensive and extensive breeding animals in Burkina Faso.
A descriptive cross-sectional study was carried out for four (4) months from March to June 2017 in the Bobo Dioulasso area.
Samples collection: we investigated stool samples collected from healthy animals (cattle = 251; pigs = 250, local poultry = 153 and exotic poultry = 244) in one (1) slaughterhouse (for cattle and pigs), five (5) livestock farms, and one (1) poultry market (for poultry) (Figure 1). For cattles and pigs, samples were collected aseptically with a swab from the colon immediately after slaughtering the animal at the abattoirs. For poultry, we made a cloacal swab. The concept of antibiotic supplementation was investigated through a questionnaire addressed to farm staff.
Isolation and identification: the swab were immediately incubated in broth heart brains. After 12-hours of incubation at 37°C, broths were subcultured on Hektoen media supplemented with 4μg/ml cefotaxim at 37°C for 24h as previously described [15,16]. Each type of colony has been purified on Hektoen media supplemented with 4μg/ml cefotaxim at 37°C for 24h and the strains were stored at -80°C until their identification by MALDI-TOF at Arnaud De Villeneuve teaching hospital at Montpellier (France). The strain E. coli ATCC 25922 was used as negative control and the strain K. pneumoniae ATCC 700603 was used as a positive control.
Susceptibility test: antimicrobial susceptibility was tested with the disk diffusion method on Müller-Hinton agar. The following antibiotics were tested: amoxicillin, amoxicillin-clavulanic acid, aztreonam, cefalexin, cefepim, cefotaxim, cefpodoxim, cefoxitin, ceftazidim, piperacillin, piperacillin-tazobactam, ticarcillin, ticarcillin-clavulanic acid, imipenem, Ertapenem, nalidixic acid, ciprofloxacin, levofloxacin, amikacin, gentamicin, tobramycin, fosfomycin, chloramphenicol, and trimethoprim-sulfamethoxazol. Results were interpreted according to the European Committee on Antimicrobial Susceptibility Testing (EUCAST) clinical breakpoints (Version 5.0) [17]. Extended-spectrum β-lactamase production was detected by using the combined double-disk synergy method [18]. For this purpose, Cefepim disc and Ticarcillin + Clavulanic acid disc were used because of the possibility of associated cephalosporinase production. Deoxyribonucleic acid (DNA) was extracted from one single colony for each isolate in a final volume of 100 μL of distilled water by incubation at 95°C for 10 min followed by a centrifugation step. The presence of, blaCTX-M (CTX-M group 1, 2, 8, 9, and 25), blaTEM, blaSHV, and blaOXA-1-like genes was assessed by multiplex PCR according to a previously published method [19]. Deoxyribonucleic acid from reference blaCTX-M, blaTEM, blaSHV-positive strains were used as positives controls. E. coli phylogenetic grouping was performed using the PCR-based method described by Clermont et al. [20]. Polymerase chain reaction products were visualized after electrophoresis on 1.5% agarose gels containing ethidium bromide at 100 V for 90 minutes. A 100 bp Deoxyribonucleic acid ladder (Promega, USA) was used as a marker size. Polymerase chain reaction products were purified using the ExoSAP-IT purification kit (GE Healthcare, Piscataway, NJ, USA) and sequenced bi-directionally on a 3100 ABI Prism Genetic Analyzer (Applied Biosystems). Nucleotide sequence alignment and analyses were performed online using the BLAST program available at the National Center for Biotechnology Information [21].
Statistical analysis: data were analyzed with Stata/IC version 13.0. The difference in the proportion of ESBL-producers between poultry groups was assessed using the chi-square test. A p-value < 0.05 was considered statistically significant.
Ethics approval and consent to participate: Burkina Faso does not have an ethics committee for animal studies, so we obtained written permission from the provincial department of animal resources of Houet (covering our study area) to conduct our study.
We identified three (3) enterobacterial species producing ESBL: E. coli (278/315), K. pneumoniae (36/315), and Citrobacter amalonaticus (1/315). Enterobacteriaceae producing ESBL was detected in 41.03% of cattle (103/251), 69.6% of pigs (174/250). Among the poultry, the Enterobacteriaceae producing ESBL was detected in 0.8% of poultry in intensive breeding (02/244) and 19.6% of poultry in extensive breeding (30/153) (p-value ˂10-3 ) (Table 1). The PCR detected three resistance genes carried: one ESBL (Bla CTX-M15) and two beta-lactamase genes (Bla TEM, Bla Oxa - 1 like) (Table 2). Phylogenetic groups could not be determined in all E. coli strains. The E. coli strains were distributed in nine phylogroup differents. The phylogroup A was the most represented among all the animals: 56.34% (40/71) among cattle, 58.50% (86/147) among pigs, and 60.71% (17/28) among poultry (Table 2).
The notion of antibiotics supplementation: this notion was found among poultry extensive and intensive breeding.
Antibiotic susceptibility patterns of Enterobacteriaceae isolates: the overall resistance of the isolates to antibiotics, (Figure 2), shows that resistance to amoxicillin (100%), Ceftazidim (99.05%), and Cotrimoxazol (86.39%) was high while resistance to Chloramphenicol (5.67%), Netilmicin (2.1%), and Amikacin (0.3%) was low. All the isolates were susceptibles to Ertapenem and Imipenem.
In this study, we investigated for the first time in Burkina Faso, the prevalence of ESBL in extensive and intensive breeding animals. Our findings show a high prevalence of ESBL in livestock in Burkina Faso (41.03% among cattle, 69.6% among pigs, and 19.6% among poultry in extensive breeding). E. coli was the most common enterobacterial species producing ESBL (278/315). One ESBL gene, belonging to the CTX-M-1 group, has been found in our study; it was the blaCTX-M-15 gene. This blaCTX-M-15 gene was often associated with the blaTEM gene and the BlaOxa-1-like gene. This study reiterates the finding in other studies worldwide, that have reported antibiotic resistance among bacteria especially E. coli isolated from animals [12,22,23]. Earlier studies reported that Klebsiella species and E. coli are the most species that produce ESBLs [24]. E. coli is considered an indicator, being a commensal bacterium ubiquitous in animals and capable of providing relevant hints on the spread of antibiotic resistance [25].
The ESBLs belonging to the CTX-M family of enzymes have been reported worldwide from a variety of different food-producing animals [12,26-28], and these animals are recognized as reservoirs of ESBLs producers [27]. In the majority of the surveys, blaCTX-M-15 was the most common ESBL gene detected worldwide in animals and humans [12,29,30]. This result has been also found in Burkina Faso among human clinical samples and fecal carriage [31,32]. Then E. coli strains belonged majority to phylogroup A in our study. The majority of african studies showed also a dominance of phylogroups A and B1 among healthy animals, human clinical samples, and human fecal carriage [12,31,32].
In our study, the prevalence of ESBLS was statistically higher among poultry extensive breeding than poultry intensive breeding and the notion of antibiotics supplementation was found among both. This notion of antibiotics supplementation could not be found among pigs, cattle (at slaughterhouse), and poultry (in the poultry market). Indeed, the animals intended for slaughter come from the urban livestock farms of Bobo-Dioulasso (38%) and the villages near and far on the periphery of Bobo Dioulasso (62%) [33]. There are two main poultry production systems in Burkina Faso, the extensive and the intensive system. The former, using local poultry, is well spread throughout the country. The intensive system, restricted to some peri-urban areas, uses exotic breeds. Rural poultry production is important and widely distributed among smallholder farms [34]. Despite in our study, like in several countries, cephalosporins are not used for animals, but the high prevalence of ESBL-producing bacteria remains [35,36]. This suggests that there are additional sources for the contamination with ESBL-producing bacteria in livestock [37]. Our situation may be explained by environmental contamination. Indeed, ESBL-producing bacteria may spread to the environment by waste products from human activities and animal production [38]. In Burkina Faso, extensive livestock farming is mainly practiced. In this extensive system, animals reared in partial confinement with supplementation, feed in the environment during pasturage (cattle) or their divagation (pigs, poultry) [33,34,39,40].
In this study, the antibiotics more used in supplementation were oxytetracyclin (Cyclin) and colistin. In Burkina Faso, antimicrobials are used in livestock in four areas: therapeutics, metaphylaxis, prophylaxis or prevention, and as a growth factor. A study conducted in 2016 found that 93.65% of farms used antimicrobials as growth promoters [41]. This practice, forbidden in Europe and North America, is also widespread in Africa [42-44]. In addition to their use as supplements, antibiotics are used as self-medication by 74.60% of livestock farmers in Burkina Faso [41]. And according to the evolution of clinical signs, 69.84% of farmers then use technical agents for veterinary care of the animals [41]. Gram positive bacteria, yeast, and fungi were not considered in this study. We were unable to determine the multi locus sequencing typing (MLST), conjugation experiment, and the supports of resistance genes.
This study has revealed, for the first time, the high prevalence of fecal carriage of CTX - M -15 ESBL among pigs, cattle, and poultry in breeding in Burkina Faso. This study strongly indicates the urgent need to establish integrated national programs of surveillance of antimicrobial use and occurrence of ESBL-producing bacteria and other antimicrobial-resistant bacteria (with zoonotic potential) among people, livestock, and the environment in Burkina Faso.
What is known about this topic
- Multidrug resistant bacteria are circulating among livestock in Africa;
- E. coli and K. pneumoniae are the main multidrug resistant bacteria found in livestock;
- Antibiotics are widely used in livestock in Africa without respect for standards and regulations.
What this study adds
- The prevalence of MDR bacteria carriage in livestock in Burkina Faso is determined for the first time;
- The source of contamination of African livestock with MDR bacteria is probably the environment.
The authors declare no competing interests.
SS, ASO, SG and JPH, conceived and designed the experiments. SS and AZ collected the samples and done the preliminary experiments. SS, ML and OOM realized the experiments. SS, AP, JZ, RT/O, ASO, GAO, JPH and SG Contributed to the writing of the manuscript. All authors read and approved the final manuscript.
We express sincere gratitude to all the individuals of slaughterhouse, the veterinary services of Bobo dioulasso, and Mr Rouamba Jeremi for their support during the study. We also acknowledge all the bacteriology laboratory actors of Arnaud de Villeneuve hospital at Montpellier, France.
Table 1: distribution of Enterobacteriaceae producing ESBL carried by animals
Table 2: analysis of antibiotic resistance genes carried and distribution of E. coli phylogroups
Figure 1: mapping of samples
Figure 2: susceptibility patterns of Enterobacteriaceae isolates of animals on the classes of antibiotics tested
- World Health Organization. Antimicrobial resistance: global report on surveillance. World Health Organization, 2014. Accessed May 24, 2018.
- Tacconelli E, Carrara E, Savoldi A, Harbarth S, Mendelson M, Monnet DL et al. Discovery, research, and development of new antibiotics: the WHO priority list of antibiotic-resistant bacteria and tuberculosis. The Lancet. Infectious diseases. 2018;18(3):318-327. PubMed | Google Scholar
- World Health Organization. The evolving threat of antimicrobial resistance: options for action. Geneva: World Health Organization, 2012. Accessed May 25, 2018.
- Laxminarayan R, Duse A, Wattal C, Zaidi AK, Wertheim HF, Sumpradit N et al. Antibiotic resistance - the need for global solutions. The Lancet Infectious Diseases. 2013 Dec;13(12):1057-98. PubMed | Google Scholar
- Landers T, Cohen B, Wittum T, Larson E. Review of antibiotic use in food animals: perspective, policy, and potential. Public Health Reports. 2012;127(1):4-22. PubMed | Google Scholar
- O´Niell J. Antimicrobials in agriculture and the environment: reducing unnecessary use and waste. In: the review on antimicrobial resistance. London: HM Government and the Wellcome Trust. 2015.
- Bell BG, Schellevis F, Stobberingh E, Goossens H, Pringle M. A systematic review and meta-analysis of the effects of antibiotic consumption on antibiotic resistance. BMC Infectious Diseases. 2014;14:13. PubMed | Google Scholar
- Voss A, Loeffen F, Bakker J, Klaassen C, Wulf M. Methicillin-resistant Staphylococcus aureus in pig farming. Emerging Infectious Diseases. 2005 Dec;11(12):1965-6. PubMed | Google Scholar
- Baptiste K, Williams K, Willams N, Wattret A, Clegg P, Dawson S et al. Methicillin-resistant Staphylococci in companion animals. Emerging Infectious Diseases. 2005 Dec;11(12):1942-4 PubMed | Google Scholar
- Alonso CA, Zarazaga M, Ben Sallem R, Jouini A, Ben Slama K, Torres C. Antibiotic resistance in Escherichia coli in husbandry animals: the African perspective. Letters in Applied Microbiology. 2017 May;64(5):318-334. PubMed | Google Scholar
- FAO. Sources of meat. 2014. Accessed May 26, 2018.
- Paterson DL, Bonomo RA. Extended-spectrum bêta lactamases: a clinical update. Clinical Microbiology Reviews. 2005;18(4):657-686. PubMed | Google Scholar
- Pitout JD, Laupland KB. Extended-spectrum betalactamase-producing Enterobacteriaceae: an emerging public health concern. The Lancet Infectious Diseases. 2008;8(3):159-166. PubMed
- Founou LL, Founou RC, Allam M, Ismail A, Djoko CF, Essack SY. Genome sequencing of extended-spectrum β-Lactamase (ESBL)-producing Klebsiella pneumoniae isolated frompigs and Abattoir Workers in Cameroon. Front. Microbiol. 2018;9:188. PubMed | Google Scholar
- Mir RA, Weppelmann TA, Johnson JA, Archer D, Morris JG Jr, Jeong KC. Identification and characterization of cefotaxime resistant bacteria in beef cattle. PLoS ONE. 2016;11(9):e0163279. PubMed | Google Scholar
- Aberkane S, Compain F, Decré D, Dupont C, Laurens C, Vittecoq M et al. High prevalence of SXT/R391-related integrative and conjugative elements carrying blaCMY-2 in Proteus mirabilis isolates from gulls in the south of France. Antimicrob Agents Chemother. 2016;60:1148-1152. PubMed | Google Scholar
- European Committee on Antimicrobial Susceptibility Testing (EUCAST). Clinical breakpoint and dosing of antibiotics, 2016. Accessed July 18, 2016.
- Jarlier V, Nicolas MH, Fournier G, Philippon A. Extended broad-spectrum beta-lactamases conferring transferable resistance to newer beta-lactam agents in Enterobacteriaceae: hospital prevalence and susceptibility patterns. Rev Infect Dis. 1988;10(4):867-878. PubMed | Google Scholar
- Dallenne CA, Da Costa, Decre C Favier G Arlet. Development of a set of multiplex PCR assays for the detection of genes encoding important beta-lactamases in Enterobacteriaceae. J Antimicrob Chemother. 2010 Mar;65(3):490-5. PubMed | Google Scholar
- Clermont O, Christenson JK, Denamur E, Gordon DM. The Clermont Escherichia coli phylotyping method revisited: improvement of specificity and detection of new phylogroups. Environ Microbiol Rep. 2013;5(1):58-65. PubMed | Google Scholar
- National library of science. Basic local alignment search tool. Accessed September 8, 2016.
- Yassin AK, Gong J, Kelly P, Lu G, Guardabassi L, Wei L et al. Antimicrobial resistance in clinical Escherichia coli isolates from poultry and livestock, China. PLoS ONE. 2017;12(9): e0185326. PubMed | Google Scholar
- Nguyen TN, Nguyen VC, Thwaites G, Carrique-Mas J. Antimicrobial usage and antimicrobial resistance in animal production in Southeast Asia: a review. Antibiotics. 2016 Nov 2;5(4):37. PubMed | Google Scholar
- Lautenbach E, Patel JB, Bilker WB, Edelstein PH, Fishman NO. Extended spectrum-lactamase-producing Escherichia coli and Klebsiella pneumoniae: risk factors for infection and impact of resistance on outcomes. Clin Infect Dis. 2001;32(8):1162-1171. Google Scholar
- CDC. European Food Safety Authority and European Centre for Disease Prevention and Control. EFSA . 2015.
- Duan RS, Sit TH, Wong SS, Wong RC, Chow KH, Mak GC et al. Escherichia coli producing CTX-M β-lactamases in food animals in Hong Kong. Microb Drug Resist. 2006;12(2):145-8. PubMed | Google Scholar
- Liu JH, Wei SY, Ma JY, Zeng ZL, Lü DH, Yang GX et al. Detection and characterisation of CTX-M and CMY-2 beta-lactamases among Escherichia coli isolates from farm animals in Guangdong Province of China. International Journal of Antimicrobial Agents. 2007;29(5):576-581. PubMed | Google Scholar
- Madec JY, Lazizzera C, Châtre P, Meunier D. Prevalence of fecal carriage of acquired expanded-spectrum cephalosporin resistance in Enterobacteriaceae strains from cattle in France. J Clin Microbiol. 2008 Apr;46(4):1566-7. PubMed | Google Scholar
- Ludden C, Raven K E, Jamrozy D, Gouliouris T, Blane B, Coll F et al. One Health genomic surveillance of Escherichia coli demonstrates distinct lineages and mobile genetic elements in isolates from humans versus livestock. BMC. 2019 Jan 22;10(1):e02693-18. PubMed | Google Scholar
- D´Andrea MM, Arena F, Pallecchi L, Rossolini GM. CTX-M-type-lactamases: a successful story of antibiotic resistance. International Journal of Medical Microbiology. 2013 Aug;303(6-7):305-17. PubMed | Google Scholar
- Ouedraogo AS, Sanou M, Kissou A, Sanou S, Somlare H, Kabore F et al. High prevalence of extended-spectrum β-lactamase producing Enterobacteriaceae among clinical isolates in Burkina Faso. BMC Infect Dis. 2016 Jul 11;16:326. PubMed | Google Scholar
- Ouedraogo AS, Sanou S, Kissou A, Poda A, Aberkane S, Bouzinbi N et al. Fecal carriage of Enterobacteriaceae producing extended-spectrum β-lactamases in hospitalized patients and healthy community volunteers in Burkina Faso. Microbial Drug Resistance. 2017;23(1):63-70. PubMed | Google Scholar
- Kindrebeogo T, Mopate LY, Kondombo SR, Kabore ZC. Approvisionnement en porcs vifs et viande porcine de la ville de Bobo-Dioulasso (Burkina Faso). Journal of Agriculture. 2012;106(2):105-122. PubMed | Google Scholar
- Pousga S, Boly H, Lindberg JE, Ogle B. Scavenging chickens in Burkina Faso: effect of season, location and breed on feed and nutrient intake. Tropical Animal Health and Production. 2005 Nov;37(8):623-34. PubMed | Google Scholar
- Smet A, Martel A, Persoons D, Dewulf J, Heyndrickx M, Catry B et al. Diversity of extended-spectrum beta-lactamases and class C beta-lactamases among cloacal Escherichia coli isolates in Belgian broiler farms. Antimicrobial Agents and Chemotherapy. 2008 Apr;52(4):1238-43. PubMed | Google Scholar
- SWEDRES-SVARM 2012. Use of antimicrobials and occur rence of antimicrobial resistance in Sweden. Solna/Uppsala ISSN 1650-6332.
- Hiroi M, Matsui S, Kubo R, Iida N, Noda Y, Kanda T et al. Factors for occurrence of extended-spectrum beta-lactamase-producing Escherichia coli in broilers. Journal of Veterinary Medical Science. 2012 Dec;74(12):1635-7. PubMed | Google Scholar
- Apata DF. Antibiotic resistance in poultry. International Journal of Poultry Science. 2009;8(4):404-408. Google Scholar
- Bagré TS, Samandoulougou S, Traoré M, Illy D, BsadjoTchamba G, Bawa-Ibrahim H et al. Détection biologique des résidus d´antibiotiques dans le lait et produits laitiers de vache consommés à Ouagadougou, Burkina Faso. J Appl Biosci. 2014;87:8105-8112. Google Scholar
- Sanon HO, Drabo A, Sangare M, Kiendrebeogo T, Gomgnibou A. Caractérisation des pratiques d´embouche bovine dans l´Ouest du Burkina Faso. Int J Biol Chem Sci. 2014;8(2):536-550. Google Scholar
- Samandoulougou S, Ilboudo AJ, Sanon OG, Tindano K, Compaoré H, Ouédraogo A et al. Utilisation de médicaments vétérinaires en production bovine et aviaire et sécurité sanitaire des aliments d´origine animale au Burkina Faso. IJMS. 2016;1:50-79.
- Adebowale O, Adeyemo OK, Awoyomi O, Dada R, Adebowale O. Antibiotic use and practices in commercial poultry laying hens in Ogun State Nigeria. Rev Elev Med Vet Pays Trop. 2016;69(1):41-45. Google Scholar
- Ben-Mahdi, MH, Ouslimani S. Mise en évidence de résidus d´antibiotiques dans le lait de vache produit dans l´Algérois. European Journal of Scientific Research. 2009;36:357-362. Google Scholar
- Takele B, Berihun T. Rational veterinary drugs use: its significance in public health. Journal of Veterinary Medecine and Animals Health. 2014;6(12):302-308. Google Scholar