Antimicrobial resistance among commensal enteric bacteria isolated from healthy cattle in Libya
Nariman Farag Almshawt, Murad Ali Hiblu, Ahmed Shaban Abid, Mohamed Salah Abbassi, Ahmed Asaid Elkady, Yousef Mohamed Abouzeed, Mohamed Omar Ahmed
Corresponding author: Mohamed Omar Ahmed, Department of Microbiology and Parasitology, Faculty of Veterinary Medicine, University of Tripoli, Tripoli, Libya
Received: 13 Mar 2020 - Accepted: 19 Mar 2020 - Published: 01 Apr 2020
Domain: Epidemiology,Veterinary medicine,Global health
Keywords: Healthy cattle, commensals bacteria, E. coli, enterococcus species, multidrug resistance, integron classes, Libya
©Nariman Farag Almshawt et al. PAMJ-One Health (ISSN: 2707-2800). This is an Open Access article distributed under the terms of the Creative Commons Attribution International 4.0 License (https://creativecommons.org/licenses/by/4.0/), which permits unrestricted use, distribution, and reproduction in any medium, provided the original work is properly cited.
Cite this article: Nariman Farag Almshawt et al. Antimicrobial resistance among commensal enteric bacteria isolated from healthy cattle in Libya. PAMJ-One Health. 2020;1:3. [doi: 10.11604/pamj-oh.2020.1.3.22325]
Available online at: https://www.one-health.panafrican-med-journal.com/content/article/1/3/full
Research 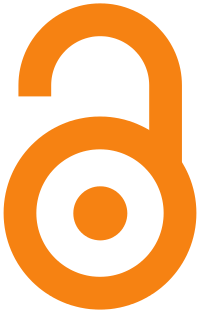
Antimicrobial resistance among commensal enteric bacteria isolated from healthy cattle in Libya
Antimicrobial resistance among commensal enteric bacteria isolated from healthy cattle in Libya
Nariman Farag Almshawt1, Murad Ali Hiblu2, Ahmed Shaban Abid3, Mohamed Salah Abbassi4, Ahmed Asaid Elkady5, Yousef Mohamed Abouzeed1, Mohamed Omar Ahmed1,&
1Department of Microbiology and Parasitology, Faculty of Veterinary Medicine, University of Tripoli, Tripoli, Libya, 2Department of Internal Medicine, Faculty of Veterinary Medicine, University of Tripoli, Tripoli, Libya, 3National Centre for Disease Control, Tripoli, Libya, 4University of Tunis El Manar, Institute of Veterinary Research of Tunisia, 20 Street Jebel Lakhdhar, Bab Saadoun, Tunis 1006, Tunisia, 5Department of Physiology and Biochemistry, Faculty of Veterinary Medicine, University of Azzaytuna, Tarhuna, Libya
&Corresponding author
Mohamed Omar Ahmed, Department of Microbiology and Parasitology, Faculty of Veterinary Medicine, University of Tripoli, Tripoli, Libya
Introduction: Enterococcus and E. coli are important commensals bacteria widely used as bio-indicators to monitor the occurrence and distribution of antimicrobial resistance.
Methods: rectal faecal samples were collected from healthy cattle from the suburban and farming areas in Tripoli within the period 2017-2018. Samples were subjected to standard and presumptive laboratory methods to isolate E. coli isolates and enterococci species then further subjected to Kirby-Bauer disk diffusion antimicrobial susceptibility test. A selection of multidrug resistant isolates of Enterococci and E. coli isolates were further investigated and analysed using the phoenix automated microbiology identification and susceptibility system. PCRs protocols were further applied to screen for blaTEM, blaSHV, bla CTX-M, class 1 and 2 integrons and the qacEΔ1-sul1 region of class 1 integrons.
Results: a total of 103 healthy cattle were included in the study yielding 206 Enterococcus species and 162 E. coli antimicrobial resistant isolates. Of these, 73% and 61% were respectively characterized as multidrug-resistant strains. The MDR enterococci strains (n=27) expressed various susceptibility patterns distributed over four different enterococci species including E. faecium, E. faecalis, E. hirae, and VanC type enterococci. Eight enterococci isolates expressed low level susceptibility to vancomycin of which three isolates were VanC intrinsic type and five were vancomycin intermediate susceptibility represented by VanC/type (n=2), E. faecium (n=1), E. hirae (n=1) and E. faecalis (n=1). The investigated MDR E. coli strains (n=61) revealed high-level resistance to ampicillin (100%), trimethoprim/sulfamethoxazole (81%), gentamicin (30%) and ciprofloxacin (7%) but no resistance was identified to other important critical antimicrobial classes. PCRs revealed that only seventeen MDR E. coli isolates (n=17/25) were positive for blaTEM, and sixteen isolates (n=16/25) positive for the class 1 integrons.
Conclusion: this is the first surveillance report on antimicrobial resistance among commensal bacterial organisms isolated from cattle in Libya. Healthy cattle can carry important bacterial strains expressing different and important antimicrobial resistance phenotypes that require continuous monitoring and antimicrobial stewardship in veterinary medicine.
Drug-resistant bacteria increasingly reported from humans, food resources and livestock including asymptomatic animals [1-8]. These are increasingly reported in the underdeveloped countries, mainly attributed to the use of antimicrobials, intensive farming production, unregulated use of antibiotics, socioeconomic factors, poor sanitation and inadequate information on antimicrobial consumption [9-13]. Food animal bacteria have major implications on public health and associated with treatment failures causing serious opportunistic and hospital acquired infections [14,15]. Commensals bacteria from food animals are exposed to antimicrobial pressure and can be a source of multidrug resistant (MDR) bacteria such as extended spectrum β-lactamases (ESBLs)-producing Enterobacteriaceae as well as vancomycin resistant enterococci [16-20]. E. coli and Enterococcus spp. are classically bio-indicator commensal organisms used to monitor antimicrobial resistance (AMR) providing essential data on the selective pressures from antimicrobial use [21-24]. In cattle, commensal E. coli isolates may serve as important reservoirs of drug resistance determinants mainly carried by mobile genetic elements, such as transposons and conjugative plasmids as well as integrons [25]. Although, the intestinal commensals of healthy population may harbour antimicrobial resistance genes that are associated with extraintestinal infections, such information particularly of animal origins are very limited [26]. The current study investigated and analysed antimicrobial resistance of commensals enterococci and E. coli strains isolated from faecal samples of healthy cattle in Tripoli between 2017-2018.
Isolation and identification of E. coli and Enterococcus spp: a fresh faecal rectal sample was obtained from each cattle and processed in the laboratory within 4 hours. Around 2-3 grams of faeces were added to 10 ml Brain Heart infusion broth (BHI) (LAP M) containing 5% glycerol and homogenized using vortex mixer. The mix was then used to streak selective mediums to characterize presumptive colonies of enterococci and E. coli. For enterococci, 1 ml from the faecal mix was added to 3 ml Kanamycin aesculin azide broth (KAAB) (LAP M) and incubated under aerobic condition at 37 oC for 24 hours. A loopful from KAAB was streaked onto Kanamycin aesculin azide agar (KAAA) and incubated under aerobic conditions at 35 oC for 48h. Plates were checked for white or grey typical colonies surrounded by black zones and further subjected for presumptive identification by Gram stains, catalase reactivity and tested for esculin hydrolysis. For E. coli, 1 ml from the faecal mix was added to 3 ml of Brilliant Green broth (Oxoid, UK) and incubated at 37 oC for 24 hours under aerobic conditions. Broths were streaked onto eosin methylene blue (EMB) agar (Oxoid, UK) and incubated under the same previous conditions. Plates were then examined for typical and presumptive round colonies of E. coli with a metallic sheen. Colonies were subjected for presumptive characterization using Gram stain, catalase and oxidase productivity and lactose fermentation. In house enterococci and E. coli strains were used as positive controls in the selection process. Two presumptive isolates of each bacterium were selected from each faecal sample and further tested for susceptibility to antimicrobial drugs.
Antimicrobial susceptibility testing: isolates were subjected to Kirby-Bauer disc diffusion method following the clinical and laboratory standards Institute guidelines [27]. E. coli strains were tested to ampicillin, tetracycline, trimethoprim/sulfamethoxazole, chloramphenicol, and nalidixic acid. Enterococci isolates were tested to ampicillin, ciprofloxacin, erythromycin, tetracycline, chloramphenicol, and gentamicin. For the purpose of the current study, isolates were identified as antimicrobial resistant (AMR) strains based on the criteria of “resistance to at least one antimicrobial agent” and as multidrug resistant (MDR) strains if the criteria of “resistance to at least two different antimicrobial agents” was characterized. Epi Info™ of the Centers for Disease Control and Prevention was used to calculate the statistically significant differences of antimicrobial resistance phenotype between the studied strains using the Chi-square test (P≤0.05). A selection of MDR isolates of each bacterium were chosen based on the inclusion of ampicillin resistance within their MDR profiles for further investigation using the phoenix automated microbiology identification and susceptibility testing system (BD Phoenix (PAMS, MSBD Biosciences, Sparks Md, US). This step was used to confirm the studied selections at species level as well as determining the susceptibility to extended antimicrobial classes. In relation to enterococci, the automated system cannot fully identify and differentiate between the intrinsic VanC type of enterococci (i.e. E. casseliflavus/gallinarum), therefore, these bacteria are referred to as VanC type enterococci in results and discussion sections.
Molecular investigation of MDR selected E. coli isolates: a selection of MDR E. coli isolates, that were fully characterized by the automated system, were chosen based on variable and extended AMR profiles and screened by PCRs for the presence of the important and common resistant genetic mechanisms; blaTEM, blaSHV, bla CTX-M, class 1 and 2 integrons and the qacEΔ1-sul1 region of class 1 integrons [28-30]. DNA was extracted by boiling a suspension of overnight- grown cultured bacterial cells in 200 μl of sterile distilled water. Each PCR mix (25 μl) contained 12.5μl HotStarTaq® Master Mix (QIAGEN, France) and 0.5μM of each primer. All PCRs were performed using an Eppendorf thermal cycler, and each run included a negative and positive inhouse control. After electrophoresis, PCR products (20 μl) were resolved on a 2% (wt/vol) agarose gels containing ethidium bromide (0.5 µg/mL). A 100-bp DNA marker (InvitrogenTM 100 bp DNA Ladder) was used to determine amplicon size.
In total, 103 healthy cattle were included originated from six dairy farms. Of these, 100% (n=103) of faecal samples were positive for at least one antimicrobial resistant enterococci species and 93% (n=96) were positive for at least one antimicrobial resistant E. coli strains. Also, 57% (59/103) of faecal samples were positive for each multidrug (MDR) Enterococcus and E. coli isolates. The process yielded a total of 206 enterococci species and 162 E. coli isolates expressing antimicrobial resistance to different antimicrobial agents. No significant differences of antimicrobial resistance expressions were identified between both bacteria for the studied and compared antimicrobial classes, except for tetracycline (P=0.00001; OR=0.3). In addition, 73% (n= 151/206) of enterococci species and 61% (n=99/162) of E. coli isolates expressed multidrug resistance (MDR) phenotypes revealing significantly higher detection rate of MDR among the studied Enterococcus species (Table 1). A selection of 27 MDR enterococci isolates were further analysed by the automated microbiological system and found to be distributed over four enterococci species; E. faecium (n=15), E. hirae (n=5), VanC/type (n=5) and E. faecalis (n=2). All isolates were susceptible to ampicillin, amoxicillin-clavulanate, ciprofloxacin, teicoplanin, nitrofurazone, erythromycin, daptomycin, mupirocin and linezolid. All isolates were resistant to trimethoprim-sulfamethoxazole, clindamycin, gentamicin, fusidic acid and cephalosporins (i.e. cefoxitin and cefotaxime) (Table 2).
Three isolates were indicated by the automated system as VRE (i.e. VanC intrinsic resistant enterococci) and five were indicated as vancomycin intermediate susceptible represented by VanC/type (n=2), E. faecium (n=1), E. hirae (n=1), and E. faecalis (n=1). Also, five enterococci were intermediately susceptible to ciprofloxacin (MICs=2 μg/mL) represented by E. faecium (n=4) and E. faecalis (n=1). A selection of 61 MDR E. coli isolates were subjected to the phoenix automated system of which 41% (n=25/61) expressed resistance to various antibiotic classes including ampicillin (100%), trimethoprim/sulfamethoxazole (81%), gentamicin (30%), and ciprofloxacin (7%). Only one isolate was identified to be resistant to cephalothin, cefuroxime, ceftriaxone, intermediate to cefepime and aztreonam but susceptible to cefoxitin and ceftazidime. Also, three strains showed intermediate susceptibility to amoxicillin-clavulanic acid. None of the MDR E. coli strains expressed resistance to amoxicillin-clavulanic acid, carbapenems (i.e. ertapenem, imipenem, meropenem), nitrofurazone, polymyxin B and colistin. The MDR E. coli isolates were further tested by PCRs revealing that seventeen MDR E. coli isolates (n=17/25) were positive for blaTEM gene, sixteen (n=16/25) isolates were positive for the intI1 gene. None of the isolates presented the blaSHV, blaCTX-M or the intI2 gene and the VR of the class 1 integron was detected only in four isolates (Table 3).
Farm animals are exposed to variable quantities of antimicrobials causing permanent alteration in the commensal microbiota [2,4,13,31]. This increases antimicrobial resistance rate up to five folds including to the same used antibiotic classes, as well as increasing the dissemination of MDR- and AMR- encoding genetic determinants [32-34]. In the current study, 93% to 100% and 57% of the studied healthy cattle were positive respectively for faecal AMR and MDR resistant strains. No significant difference was observed between both of the commensal bacterium in the resistance shown to different antimicrobial agents, however; enterococci expressed higher MDR phenotypes comparing to E. coli. In the current study, the 27 studied MDR enterococci isolates were found to belong to four different species mainly E. faecium. The E. faecium strains expressed resistance only to trimethoprim-sulfamethoxazole, clindamycin, gentamicin, fusidic acid and cephalosporins (i.e. cefoxitin and cefotaxime). In addition, five enterococci strains were identified as vancomycin intermediate susceptibility (MICs=8μg/mL) represented by VanC/type enterococci (n=2), E. faecium (n=1), E. hirae, (n=1) and E. faecalis (n=1). This corresponds with a recent pan-surveillance survey from the EU investigating the susceptibility of large collection of commensal enterococci collected from food-producing animals [24]. This report revealed variable antibiotic susceptibility and low to absent resistance to the critically important antimicrobial such as ampicillin, gentamicin, linezolid, tigecycline and vancomycin, but high resistance to quinupristin/dalfopristin, tetracycline and erythromycin [24]. This recent survey revealed similar results to our current study in regards the decreased susceptibility to vancomycin (i.e. MICs of 8μg/mL) only among few enterococci species.
In food production, the emergence of different VRE genotypes, of animal origins (e.g. cattle, poultry) has been mainly linked to the widespread use of sub-therapeutic antibiotics [35]. The VanA-VRE type is frequently associated with high level of resistance to both vancomycin (MIC≥64μg/mL) and teicoplanin (MIC≥16μg/mL), whereas VanB is associated with varying levels of resistance to vancomycin (MIC 1-1000 μg/mL) but not to teicoplanin [20,35]. A study investigating VRE strains which were identified based on criteria of MICs≥8mg/L using an automated identification system, has reported vanC-1 resistance gene in E. faecium and E. gallinarum characterized by low-level resistance to vancomycin [36]. Another study analysing clinical and faecal strains of 60 VRE/vancomycin intermediate enterococci (i.e. MIC of 8-16 μg/mL), has identified vanA or vanB genes in E. faecalis and E. faecium strains, but only vanB gene in E. gallinarum strains [37]. The VanC types (i.e. E. casseliflavus/E. gallinarum) has attracted less attention, despite their increasing associations with human infections and outbreaks; however, these inducible types are associated with antibiotic exposure able to express various vancomycin resistant phenotypes such as VanA, VanB and VanD types [20]. In the current study, only blaTEM and class 1 integrons were identified respectively among 17 and 16 isolates, of the 25 studied MDR E. coli isolates. These findings correspond with global trends on the most reported genetic mechanisms responsible for MDR phenotypes [29]. A recent report has documented antimicrobial-resistant Gram-negative bacteria from 81% of 102 fecal rectal samples of healthy human population mainly expressing β-lactamase genes (blaTEM, blaSHV, blaCTX-M, and blaOXA) (72%) and Integron sequences (36%) [26]. Another study from North Africa investigating 174 E. coli isolates collected from healthy food producing animals including cattle reported MDR phenotypes in 44.2% of the collection but the absence of ESBL producers [9].
Class 1 integrons are mainly reported in MDR E. coli isolates in cattle however, class 2 integrons are reported at low frequencies [38,39]. Class 1 integrons are widely reported from different animals carried by various bacterial organisms such as E. coli, K. pneumoniae and S. aureus and associated with both vertical and horizontal transmission [40,41]. Recent reports have shown that E. coli isolates from healthy cattle and non-healthy calves can carry highly diverse gene cassettes encoding antibiotic resistance in the variable regions of their class 1 integrons such as flo, dfrA, and aadA gene cassette [42,43]. Class 1 integron has been particularly associated with various alleles of dfr-encoding trimethoprim resistance (e.g. dfrA1 and dfrA17) and tetracycline resistance genes in commensal E. coli isolated from cattle and associated with antimicrobial pressure including at the subtherapeutic doses of sulfamethazine and/or chlortetracycline [44,45]. In the present study, the presumptive isolation process used in the current study have been widely documented as reliable laboratory protocols for reliable characterization of commensals E. coli and enterococci species [46,47]. The automated identification systems (including the phoenix system) have been increasingly used showing accurate performance for the detection and analysis of AMR and MDR pathogens (e.g. ESBLs and VRE) [48-50].
This is the first study that provides novel data on the status and distribution of antimicrobial resistance in food producing animals in Libya. The gastrointestinal microbiota of cattle may harbour commensal bacterial species carrying various antibiotic resistance determinants of public health concerns particularly among the enterococci species. Antimicrobial stewardship in animal medicine is very important to overcome the critical consequences of AMR and MDR on human health and further investigations and monitoring studies are required.
What is known about this topic
- Antimicrobial resistance in a global serious concern affecting humans and animals with variable geographic distribution;
- Animals particularly food-producing livestock play important role in the dissemination and development of antimicrobial resistance;
- The available data are mostly related to humans and hospital acquired bacteria with little information of zoonotic origins.
What this study adds
- The important and possible role of commensals as a reservoir of antimicrobial resistance and the importance of continuous monitoring;
- Understand the burden of bacterial drug resistance and pressure effect of antimicrobials use in important food animals;
- Data and epidemiological information particularly of zoonotic nature on the spread of drug resistant bacteria in food animals in North African region.
Authors declare no conflicts of interests.
Almshawt NF collected samples, presented data and performed laboratory analysis. Hiblu MA has contributed in collecting samples and data. Abid AS and Abbassi MS have equally contributed in the laboratory work and interpretation of results. Abouzeed YM and Elkady AA have equally contributed in presenting and analysing data. Ahmed MO has contributed in designing the study, interpretation of results and writing the manuscript.
Authors are thankful to our colleagues at national centre for disease control and the animal health centre in Tripoli, Libya for their support and encouragement.
Table 1: susceptibility to antimicrobials of Enterococcus and E. coli resistant isolates
Table 2: summary and distribution of antibiotic resistance of MDR Enterococcus species isolated from faecal samples
Table 3: the MICs values and interpretation of MDR E. coli isolates and the associated genes and genetic determinants (BD Phoenix) (CLSI)
- World Health Organization. Critically important antimicrobials for human medicine, 6th revision. WHO Advisory Group on Integrated Surveillance of Antimicrobial Resistance (AGISAR). 2019. Google Scholar
- Hoelzer K, Wong N, Thomas J, Talkington K, Jungman E, Coukell A. Antimicrobial drug use in food-producing animals and associated human health risks: what, and how strong, is the evidence. BMC Vet Res. 2017;4;13(1):211. PubMed | Google Scholar
- Economou V, Gousia P. Agriculture and food animals as a source of antimicrobial-resistant bacteria. Infect Drug Resist. 2015 Apr 1;8:49-61. PubMed | Google Scholar
- Magouras I, Carmo LP, St&aouml;rk KDC, Schüpbach-Regula G. Antimicrobial Usage and -Resistance in Livestock: Where Should We Focus. Front Vet Sci. 2017;15;4:148. PubMed | Google Scholar
- K&oouml;ck R, Cuny C. [Multidrug-resistant bacteria in animals and humans]. Europe PMC. 2018. Google Scholar
- Geser N, Stephan R, H&aouml;chler H. Occurrence and characteristics of extended-spectrum β-lactamase (ESBL) producing Enterobacteriaceae in food producing animals, minced meat and raw milk. BMC Vet Res. 2012;8:21. PubMed | Google Scholar
- Ahmed MO, Aghila E, Elamri S, Baptiste KE. Molecular investigation of carbapenemase-producing Enterobacteriaceae isolated from a Tripoli Hospital, Libya. Libyan J Med Sci. 2017;1:80-82. Google Scholar
- Kieffer N, Ahmed MO, Elramalli AK, Daw MA, Poirel L, Alvarez R et al. Colistin-resistant carbapenemase-producing isolates among Klebsiella spp. and Acinetobacter baumannii in Tripoli, Libya. J Glob Antimicrob Resist. 2018;13:37-39. PubMed | Google Scholar
- Abbassi MS, Kilani H, Zouari M, Mansouri R, Oussama EF, Hammami S et al. Antimicrobial Resistance in Escherichia Coli Isolates from Healthy Poultry, Bovine and Ovine in Tunisia: A Real Animal and Human Health Threat. J Clin Microbiol Biochem Technol. 2017;3(2):019-023. Google Scholar
- Maron DF, Smith TJ, Nachman KE. Restrictions on antimicrobial use in food animal production: an international regulatory and economic survey. Global Health. 2013;9:48. PubMed | Google Scholar
- Manyi-Loh C, Mamphweli S, Meyer E, Okoh A. Antibiotic Use in Agriculture and Its Consequential Resistance in Environmental Sources: Potential Public Health Implications. Molecules. 2018;30;23(4). PubMed | Google Scholar
- da Costa PM, Loureiro L, Matos AJ. Transfer of multidrug-resistant bacteria between intermingled ecological niches: the interface between humans, animals and the environment. Int J Environ Res Public Health. 2013;14;10(1):278-294. PubMed | Google Scholar
- Marshall BM, Levy SB. Food animals and antimicrobials: Impacts on human health. Clin Microbiol Rev. 2011;24(4):718-733. PubMed | Google Scholar
- Koo HJ, Woo GJ. Distribution and transferability of tetracycline resistance determinants in Escherichia coli isolated from meat and meat products. Int J Food Microbiol. 2011;145(2-3):407-413. PubMed | Google Scholar
- Kim MC, Cha MH, Ryu JG, Woo GJ. Characterization of Vancomycin-Resistant Enterococcus faecalis and Enterococcus faecium Isolated from Fresh Produces and Human Fecal Samples. Foodborne Pathog Dis. 2017;14(4):195-201. PubMed | Google Scholar
- Szmolka A, Nagy B. Multidrug resistant commensal Escherichia coli in animals and its impact for public health. Front Microbiol. 2013; 3;4:258. PubMed | Google Scholar
- Tacconelli E, Carrara E, Savoldi A, Harbarth S, Mendelson M, Monnet DL et al. WHO Pathogens Priority List Working Group. Discovery, research, and development of new antibiotics: the WHO priority list of antibiotic-resistant bacteria and tuberculosis. Lancet Infect Dis. 2018;18(3):318-327. PubMed | Google Scholar
- Silley P, Simjee S, Schwarz S. Surveillance and monitoring of antimicrobial resistance and antibiotic consumption in humans and animals. Rev Sci Tech. 2012;31(1):105-20. PubMed | Google Scholar
- World Health Organization. Antimicrobial resistance: global antibiotic resistance surveillance report. Geneva. 2014. Google Scholar
- Ahmed MO, Baptiste KE. Vancomycin-Resistant Enterococci: A Review of Antimicrobial Resistance Mechanisms and Perspectives of Human and Animal Health. Microb Drug Resist. 2018;24(5):590-606. PubMed | Google Scholar
- Murphy D, Ricci A, Auce Z, Beechinor JG, Bergendahl H, Breathnach R et al. EMA and EFSA Joint Scientific Opinion on measures to reduce the need to use antimicrobial agents in animal husbandry in the European Union, and the resulting impacts on food safety (RONAFA). EFSA J. 2017;15:4666. Google Scholar
- Aasmäe B, Häkkinen L, Kaart T, Kalmus P. Antimicrobial resistance of Escherichia coli and Enterococcus spp isolated from Estonian cattle and swine from 2010 to 2015. Acta Vet Scand. 2019;21;61(1):5. PubMed | Google Scholar
- González-Sanz R, Herrera-León S, de la Fuente M, Arroyo M, Echeita M. Emergence of extended-spectrum β-lactamases and AmpC-type β-lactamases in human Salmonella isolated in Spain from 2001 to 2005. J Antimicrob Chemoth. 2009;64(6):1181-1186. PubMed | Google Scholar
- de Jong A, Simjee S, Rose M, Moyaert H, El Garch F, Youala M et al. Antimicrobial resistance monitoring in commensal enterococci from healthy cattle, pigs and chickens across Europe during 2004-14 (EASSA Study). J Antimicrob Chemother. 2019;74(4):921-930. PubMed | Google Scholar
- de Jong A, Bywater R, Butty P, Deroover E, Godinho K, Klein U et al. A pan-European survey of antimicrobial susceptibility towards human-use antimicrobial drugs among zoonotic and commensal enteric bacteria isolated from healthy food-producing animals. J. Antimicrob. Chemother. 2009;63(4):733-744. PubMed | Google Scholar
- Rubin J, Mussio K, Xu Y, Suh J, Riley LW. Prevalence of Antimicrobial Resistance Genes and Integrons in Commensal Gram-Negative Bacteria in a College Community. Microb Drug Resist. 2020;10. PubMed | Google Scholar
- Clinical Laboratory Standard Institution (CLSI). M100-S25 performance standards for antimicrobial susceptibility testing. Twenty-fifth informational supplement. 2015. Google Scholar
- Jouini A, Vinué L, Slama KB, Sáenz Y, Klibi N, Hammami S et al. Characterization of CTX-M and SHV extended-spectrum beta-lactamases and associated resistance genes in Escherichia coli strains of food samples in Tunisia. J Antimicrob Chemother. 2007;60(5):1137-1141. PubMed | Google Scholar
- Sáenz Y, Briñas L, Domínguez E, Ruiz J, Zarazaga M, Vila J et al. Mechanisms of resistance in multiple-antibiotic-resistant Escherichia coli strains of human, animal, and food origins. Antimicrob Agents Chemother. 2004;48(10):3996-4001. PubMed | Google Scholar
- Carattoli A, Villa L, Pezzella C, Bordi E, Visca P. Expanding drug resistance through integron acquisition by IncFI plasmids of Salmonella enterica Typhimurium. Emerg Infect Dis. 2001;7(3):444-447. PubMed | Google Scholar
- Miranda JM, Vázquez BI, Fente CA, Barros-Velázquez J, Cepeda A, Franco CM. Evolution of resistance in poultry intestinal Escherichia coli during three commonly used antimicrobial therapeutic treatments in poultry. Poult Sci. 2008;87(8):1643-1648. PubMed | Google Scholar
- Silva N, Igrejas G, Gonçalves A, Poeta P. Commensal gut bacteria: Distribution of Enterococcus species and prevalence of Escherichia coli phylogenetic groups in animals and humans in Portugal. Ann Microbiol. 2012;62: 449-459. Google Scholar
- Kissinga HD, Mwombeki F, Said K, Katakweba AAS, Nonga HE, Muhairwa AP. Antibiotic susceptibilities of indicator bacteria Escherichia coli and Enterococci spp isolated from ducks in Morogoro Municipality, Tanzania. BMC Res Notes. 2018;31;11(1):87. PubMed | Google Scholar
- Clemente JC, Ursell LK, Parfrey LW, Knight R. The impact of the gut microbiota on human health: an integrative view. Cell. 2012;16;148(6):1258-1270. PubMed | Google Scholar
- Rengaraj R, Mariappan S, Sekar U, Kamalanadhan A. Detection of Vancomycin Resistance among Enterococcus faecalis and Staphylococcus aureus. J Clin Diagn Res. 2016;10(2):DC04-6. PubMed | Google Scholar
- Kobayashi K, Rao M, Keis S, Rainey FA, Smith JM, Cook GM. Enterococci with reduced susceptibility to vancomycin in New Zealand. J Antimicrob Chemother. 2000;46(3):405-410. PubMed | Google Scholar
- Biswas PP, Dey S, Adhikari L, Sen A. Detection of vancomycin resistance in enterococcus species isolated from clinical samples and feces of colonized patients by phenotypic and genotypic methods. Indian J Pathol Microbiol. 2016;59(2):188-193. PubMed | Google Scholar
- Wu RB, Alexander TW, Li JQ, Munns K, Sharma R, McAllister TA. Prevalence and diversity of class 1 integrons and resistance genes in antimicrobial-resistant Escherichia coli originating from beef cattle administered subtherapeutic antimicrobials. J Appl Microbiol. 2011;111(2):511-523. PubMed | Google Scholar
- Gillings M, Boucher Y, Labbate M, Holmes A, Krishnan S, Holley M et al. The evolution of class 1 integrons and the rise of antibiotic resistance. J Bacteriol. 2008;190(14):5095-5100. PubMed | Google Scholar
- Mazel D. Integrons: agents of bacterial evolution. Nat. Rev. Microbiol. 2006;4(8):608-620. PubMed | Google Scholar
- Sunde M, Norström M. The genetic background for streptomycin resistance in Escherichia coli influences the distribution of MICs. J Antimicrob Chemother. 2005;56(1):87-90. PubMed | Google Scholar
- Chen CM, Ke SC, Li CR, Wu YC, Chen TH, Lai CH et al. High Diversity of Antimicrobial Resistance Genes, Class 1 Integrons, and Genotypes of Multidrug-Resistant Escherichia coli in Beef Carcasses. Microb Drug Resist. 2017;23(7):915-924. PubMed | Google Scholar
- Ahmed AM, Younis EE, Osman SA, Ishida Y, El-Khodery SA, Shimamoto T. Genetic analysis of antimicrobial resistance in Escherichia coli isolated from diarrheic neonatal calves. Vet Microbiol. 2009;136(3-4):397-402. PubMed | Google Scholar
- Díaz-Mejía JJ, Amábile-Cuevas CF, Rosas I, Souza V. An analysis of the evolutionary relationships of integron integrases, with emphasis on the prevalence of class 1 integrons in Escherichia coli isolates from clinical and environmental origins. Microbiology. 2008;154(1):94-102. PubMed | Google Scholar
- Belaynehe KM, Shin SW, Yoo HS. Interrelationship between tetracycline resistance determinants, phylogenetic group affiliation and carriage of class 1 integrons in commensal Escherichia coli isolates from cattle farms. BMC Vet Res. 2018;14(1):340. PubMed | Google Scholar
- Ahmed MO, Clegg PD, Williams NJ, Baptiste KE, Bennett M. Antimicrobial resistance in equine faecal Escherichia coli isolates from North West England. Ann Clin Microbiol Antimicrob. 2010;7;9:12. PubMed | Google Scholar
- Ahmed MO, Clegg PD, Williams NJ, Baptiste KE, Bennett M. Vancomycin resistant enterococci (VRE) in equine-faecal samples. In science and Technology against Microbial Pathogens. Research, Development and Evaluation. World Scientific Publishing. 2011; P357-362. Google Scholar
- Duggal S, Gaind R, Tandon N, Deb M, Chugh TD. Comparison of an automated system with conventional identification and antimicrobial susceptibility testing. ISRN Microbiol. 2012;107203. PubMed | Google Scholar
- Hong JS, Kim D, Kang DY, Park BY, Yang S, Yoon EJ et al. Evaluation of the BD Phoenix M50 Automated Microbiology System for Antimicrobial Susceptibility Testing with Clinical Isolates in Korea. Microb Drug Resist. 2019;25(8):1142-1148. PubMed | Google Scholar
- Brigante GR, Luzzaro FA, Pini B, Lombardi G, Sokeng G, Toniolo AQ. Drug susceptibility testing of clinical isolates of streptococci and enterococci by the Phoenix automated microbiology system. BMC Microbiol. 2007;23;7:46. PubMed | Google Scholar