Seroprevalence of Q-fever, spotted fever, typhus group Rickettsia and Orientia among febrile patients visiting hospital-based sentinel sites in Uganda: a cross-sectional study
Wilfred Eneku, Bernard Erima, Anatoli Maranda Byaruhanga, Nora Gillian Cleary, Gladys Atim, Titus Tugume, Qouilazoni Aquino Ukuli, Hannah Kibuuka, Edison Mworozi, Christina Douglas, Jeffrey William Koehler, Michael Emery von Fricken, Savino Biryomumaisho, Robert Tweyongyere, Fred Wabwire-Mangen, Denis Karuhize Byarugaba
Corresponding author: Wilfred Eneku, Makerere University, College of Veterinary Medicine, P.O. Box 7062, Kampala, Uganda
Received: 19 Aug 2023 - Accepted: 09 Sep 2023 - Published: 15 Sep 2023
Domain: Epidemiology,Community health,Vector biology
Keywords: Q-fever, murine typhus, spotted fever, zoonoses, orientia, rickettsioses, East Africa
©Wilfred Eneku et al. PAMJ-One Health (ISSN: 2707-2800). This is an Open Access article distributed under the terms of the Creative Commons Attribution International 4.0 License (https://creativecommons.org/licenses/by/4.0/), which permits unrestricted use, distribution, and reproduction in any medium, provided the original work is properly cited.
Cite this article: Wilfred Eneku et al. Seroprevalence of Q-fever, spotted fever, typhus group Rickettsia and Orientia among febrile patients visiting hospital-based sentinel sites in Uganda: a cross-sectional study. PAMJ-One Health. 2023;12:3. [doi: 10.11604/pamj-oh.2023.12.3.41475]
Available online at: https://www.one-health.panafrican-med-journal.com/content/article/12/3/full
Research 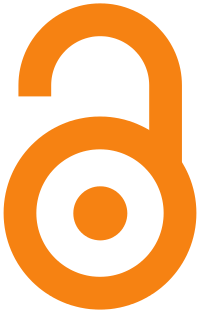
Seroprevalence of Q-fever, spotted fever, typhus group Rickettsia and Orientia among febrile patients visiting hospital-based sentinel sites in Uganda: a cross-sectional study
Seroprevalence of Q-fever, spotted fever, typhus group Rickettsia and Orientia among febrile patients visiting hospital-based sentinel sites in Uganda: a cross-sectional study
Wilfred Eneku1,2,&, Bernard Erima2, Anatoli Maranda Byaruhanga2,
Nora Gillian Cleary3,
Gladys Atim2, Titus Tugume2, Qouilazoni Aquino Ukuli2, Hannah Kibuuka2, Edison Mworozi4, Christina Douglas5,
Jeffrey William Koehler5, Michael Emery von Fricken3, Savino Biryomumaisho1, Robert Tweyongyere1, Fred Wabwire-Mangen2,6, Denis Karuhize Byarugaba1,2
&Corresponding author
Introduction: rickettsioses are emerging zoonotic febrile illnesses transmitted to humans by ticks, fleas, lice, and mites. Q-fever, Spotted fever group (SFG), Typhus group (TG) rickettsia and Scrub typhus (STG) have been reported with varying prevalence across East Africa. However, little is known about the burden of exposure in Uganda. The aim of this study was to determine the seroprevalence and associated risk factors of rickettsial diseases in Uganda.
Methods: a total of 460 archived serum samples collected from patients with fever of unknown origin after screening across five hospital-based sentinel sites were analysed. The samples were collected during 18-month period of active surveillance for acute febrile illnesses, from January 2018 through June 2019. We performed IgM ELISA tests on the 460 sera for SFG and TG rickettsia, IgM IFA for STG and Phase 2 IgG ELISA for Q-fever. We also assessed risk factors associated with the serostatus.
Results: the population comprised predominantly children, had balanced gender proportions, with 66% coming from rural areas. The overall seroprevalence of SFG rickettsiosis was 6.3%; however, 11.5% and 10.8% prevalence rates were observed in Gulu and Bwera hospitals respectively. This was higher than the 3.7% observed in the capital city Kampala, although the differences were not statistically significant (Fisher's exact = 0.489). Overall seropositivity of Q-fever was 7.6%, although Bwera Hospital had the highest rate (12.5%) and Mulago had the lowest rate (2%). The differences were not considered statistically significant (Fishers exact= 0.075). Increasing age (OR-adjusted=1.4, 95%CI=1.0-1.9, p=0.026) and rural background (OR-adjusted=2.6, 95%CI=1.6-6.4, p=0.037) were both significantly associated with seropositivity for Q-fever, while only increasing age had higher odds for seropositivity for SFG rickettsia (OR-adjusted= 1.9, 95% CI= 1.4-2.6, p<0.001). One serum sample of a 10-month-old male from Bwera hospital was reactive to both SFG and Q-fever antibodies. We found four sera reactive cases to typhus group IgM and another four reactive to Orientia spp. IgM. However, we were not able to determine associating factors due to low seropositivity rates.
Conclusion: here, we report for the first time the seroprevalence of Q-fever, SFG and STG in febrile patients in Uganda. This report also provides the second study in over five decades since the earliest report of TG rickettsia. Testing for these pathogens in patients with acute febrile illness with unknown etiology may hold value, however more studies are required to provide information on disease ecology, risk factors, and transmission dynamics of these pathogens in Uganda.
Rickettsioses are emerging zoonotic bacterial infections transmitted to humans by ticks, fleas, lice, and mites worldwide [1,2]. The causative agents are non-motile intracellular short Gram-negative rods in the order Rickettsiales. The taxonomy of rickettsia has evolved over the years with advances of molecular genetics and genomics. However, four diseases are still commonly called rickettsioses which include 1) spotted fever (SFG) and typhus group (TG) rickettsia caused by bacteria in the order Rickettsia, 2) scrub typhus group (STG) due to Orientia tsutsugamushi (formerly Rickettsia tsutsugamushi), 3) Q-fever due to Coxiella burnetii (now removed from order Rickettsiales) and 4) human ehrlichiosis and anaplasmosis from the family Anaplasmatceae [3,4]. Rickettsia typhi, the causative agent of TG rickettsia, is transmitted to humans by rodent fleas, Xenopsylla cheopis, while the over thirty SFG rickettsia are transmitted by ticks with the exception of R. felis, the agent of flea-borne spotted fever, which is vectored by cat fleas, Ctnedocephalides felis [5,6]. New pathogenic species of SFG rickettsia have been detected with increasing frequency in the past decade [7,8].
Spotted Fever Group, TG, STG rickettsioses and Q-fever often present as acute nonspecific febrile illnesses that are difficult to diagnose clinically [9,10]. The diseases are often diagnosed by molecular and serological tests that require specialized laboratory capacity and expertise which is often lacking in low resource settings [11,12]. Moreover, confirmatory diagnosis by serological tests require at least 4-folds increase in convalescent serum to IgG titers, which is often too late to start specific treatment [13,14]. PCR tests that are accurate and more specific are often expensive and may test negative from whole blood in early stages of the disease due to localized growth of organisms, intracellularly, often in endothelium of blood vessels [15]. Consequently, the importance of SFG, TG, STG and Q-fever as differentials of acute febrile illness in sub-Saharan Africa is poorly characterized. In the tropics, where the vectors are quite abundant all year round [16,17], rickettsial infections seem to play a larger role in induction of acute febrile illnesses than previously thought. Occupational exposures in abattoir workers to SFG, TG and Q-fever have been reported in Djibouti, Horn of Africa [18] and high seroprevalence rates of flea-borne typhus were detected in Australian Veterinarians [19]. By implication, a large proportion of individuals living in Sub-Saharan Africa who derive their livelihoods through agriculture and animal keeping are at risk of infection. In the East African region, these diseases have been detected in febrile patients in hospitals in Kenya [9,20] and Tanzania [13,21] with varying seroprevalences of 8-10% for SFG and 0.5-1% for TG. In the Kenyan study, scrub typhus was detected in 5% of the patients, with the highest prevalence in Marigat town located near the Ugandan eastern border [20]. Moreover, the chigger mites, the vector for STG has been proven to occur in Kenya [22]. These findings imply that STG is not limited to Asia where it originally occurred but also East Africa, though Orientia chuto could be the circulating causative agent in this region.
To date, little is known about rickettsioses in Ugandan indigenous population. The earliest detection of zoonotic rickettsia in Uganda was in 1950s when Baird and Tonkin reported the occurrence of endemic typhus in three patients in the then Mengo district [23]. Thereafter, in 1972, Sezi and others reported a small outbreak of louse-borne (epidemic) typhus in a refugee population in Masaka district in which 13 people were hospitalised with one fatality [24]. The last report of epidemic typhus fever outbreak with fatalities occurred between 1975 and 1976 in Ankole region among Rwandan refugee-herdsmen who lived in squalid conditions [25]. Since then, there has been a vacuum in knowledge of rickettsioses in indigenous populations of Uganda despite reports of acute SFG-rickettsiosis in international travellers returning from the country [26]. Furthermore, R. africae, the causative agent of African spotted fever, detected with high prevalence (97.1%) in Ambylomma variegatum [27] and R. conorii, the agent of Mediterranean spotted fever from Haemophysalis punctalaechi [28] and other SFG rickettsia in ticks have been reported in Uganda [29]. We recently reported the wide distribution of SFG rickettsia in multiple tick species in Uganda [17,30]. Rickettsia felis has also been reported in cat fleas in the country [31]. These findings suggest Rickettsia infection as potential causes of fever in the local population and travellers who get exposed.
Q-fever, caused by Coxiella burnetii, manifests with nonspecific signs such as fever, chills, fatigue, and muscle pain [32], that may be clinically mistaken for endemic febrile illnesses like malaria or mild viral diseases. The disease may become chronic in 1% of the patients and results in endocarditis which is often fatal [33]. The major mode of transmission is by inhalation of the bacterium in contaminated dust with animal excreta or birth-fluids from infected animals, particularly ruminants (cattle, goats and sheep) that act as reservoirs [34]. Transmission by tick-bites have been reported, [35], but the extent of infection caused by ticks remains poorly understood. Livestock rearing and consumption of inappropriately handled animal products are other potential risk factors of exposure. Q-fever has been reported in febrile patients of indigenous populations in Tanzania with 5% prevalence [13] but little is known about the disease in other east African countries including Uganda. The Uganda clinical guidelines in this study period placed rickettsial diseases to be managed by health centre IIs [36], which are ill-equipped to diagnose these pathogens. Therefore, the aim of this study was to determine the seroprevalence and determine the associated risk factors among febrile patients from five regional referral hospitals using the archived serum and complementary data collected alongside the samples. The hospitals are found in four different economic and cultural zones of Uganda.
Study design: this was a cross-sectional study in which samples were collected from febrile patients of unknown origin over 18-month period and archived.
Study setting and population: the study was conducted under Makerere University Walter Reed Project hospital-based sentinel sites for acute febrile illnesses surveillances. Samples were collected by health workers from febrile patients who sought treatment from the five established surveillance hospitals. These included Jinja regional referral hospital (Eastern Uganda), Mulago National referral hospital (Central Uganda), Bwera regional referral hospital (Western Uganda), Gulu regional referral hospital (Northern Uganda) and Bombo General Military hospital (Central Uganda) (Figure 1). The districts in which the hospitals are found constitute major economic hubs in the four regions, and they are geographically diverse, culturally and economically heterogeneous. The majority of patients in the particular hospital were the local populations within the districts and the neighborhoods.
Participants, sample collection and storage: febrile patients who sought healthcare services at any of the sentinel sites between January 2018 through June 2019 were enrolled into the study upon signing written consent. Patients aged 6 months and older were enrolled upon assent. Legal guardians signed on behalf of the minors, while adults signed the written consent by themselves. The inclusion criteria for pediatric patients 6 months to 13 years of age was a history of fever in the last 48 hours or measured temperature of 37.5°C (axillary) or 38°C (rectal) and above. Oral temperature = 38°C was considered for inclusion of adolescents and adults. Both out and in-patients were included. Of those who consented for enrollment, a trained medical officer collected standardized data on patient demographics, clinical history and physical examination. Individuals who had known cause of fever, such as malaria, acute sepsis and others (after the standard tests prescribed by the clinical guidelines) and those who did not consent were excluded from the study. Blood samples were drawn into anticoagulant free vacutainers and transported to the Emerging Infectious Diseases Project (EIDP) Laboratory, at Makerere University. The serum was harvested and stored at -80 °C till analysis.
Sample size: a total of 1,485 serum samples were collected in the 18-month period, of which 460 were selected in this study by stratified random sampling, with each hospital representing a stratum. The selected archived samples are those that met the minimum requirements with retrievable complementary data such as age, sex, residence and occupation. The 460 meets the minimum required sample size at 50% assumed prevalence and 95% confidence interval for unknown prevalence of a certain disease in the population using the principle of Thrusfield in epidemiology [37]. The selected serum samples were tested for Q-fever, SFG, TG rickettsia and scrub typhus.
Laboratory testing and analysis: archived serum samples were retrieved, thawed and together with the commercial ELISA kit reagents, were allowed to equilibrate to room temperature before the start of timed test procedure. Phase 2 immunoglobulin G (IgG) enzyme-linked immunosorbent assay (ELISA) kits for Q-Fever and indirect enzyme immunoassay (EIA) kit for IgM antibodies against TG (Cat.# RTM-96K) and SFG rickettsia (Cat.# RRM-96K) from Fuller laboratories (Fullerton, California 92831, USA) were used according to the manufacturer´s recommendations. For the SFG rickettsia, EIA microwells in the kit utilize outer membrane proteins (rOmp A and rOmp B) which contain both species-specific and more broadly reactive determinants. Patient sera were then diluted in the Sample Diluent to a final dilution of 1:100, with 100µl of diluted serum and diluted controls each added into microwells in duplicate. Microwells were covered with an adhesive strip then incubated for 60 minutes at room temperature, followed by four washes with Wash Buffer to remove unreacted serum proteins, after which an enzyme-labeled anti-human IgM (enzyme, HRP-conjugate) was added to label the bound antibody. After an incubation period of 30 minutes at room temperature, the microwells were washed to remove unbound enzyme conjugate. To each microwell, 100µl of enzyme substrate (TMB substrate) was added to quantitate the bound peroxidase portion of the conjugate for 10 minutes. Blue color developed is directly proportional amount of reactive serum antibody. The reaction was stopped by adding 100µl of Stop solution and turned the blue color to yellow and stabilized the reaction. The absorbance (color intensity) was then measured at wavelength of 450nm on a microtiter plate reader.
The antigens used in the SFG detection kit were purified from Rickettsia rickettsia but cross reacts with R. montanensis, R. parkeri, R. conorii and R. africae. Typhus Group rickettsial EIA was performed with a similar procedure that detects the outer membrane protein (rOmp B) purified from R. typhi using the kit protocol. Final serum dilution of 1:100 was used and the absorbance was measured at 450nm wavelength on microtiter plate reader. The acceptance criteria calculating the absorbance ranges and ratios according to the protocol. IgM against Orientia was tested using Orientia tsutsugamushi IFA Kit OTM-100 (Fullerton, California 92831 USA). The IFA slides in the kit utilize 4 strains (Gilliam, Karp, Boryong and Kato), presented in L929 cells in a linear pattern within each slide well. First, the screening of the samples was done by diluting the patient serum to 1:128 in PBS. For each dilution, 20µl of diluted serum was added to slide well and incubated at 37°C for 30 minutes in a humidity chamber. The slides were then removed from humidity chamber and washed to remove non-reactive serum proteins. A drop (20µl) of fluorescence conjugate was added and incubated at 37°C for 30 minutes in humidity chamber to label the antigen-antibody complexes. After further incubation, the slides were washed again to remove the non-reactive conjugate and cover-slipped. The resulting reactions were visualized using a fluorescence microscopy (Nikon Eclipse E200MV RS, Nikon, Tokyo, Japan), comparing the sample reactions to the positive and negative controls. Positive samples were titrated at higher dilutions to determine end point dilution (1:512).
Statistical analysis: demographic data and laboratory results were retrieved into MS-excel spreadsheet and analyzed using Stata software, v.15 (Stata Corp). The participants´ residence were classified as rural or urban based on 2014 national census data [38]. Descriptive statistics demonstrating proportions and measures of association between categorical variables were performed using 2-sided Fisher´s exact test and logistic regression analysis.
Ethical approval and consent to participate: this study was performed under Protocol "Acute Febrile Illness in Uganda” approved by Makerere University School of Public Health Research and Ethics Committee (MakSPH-REC #369), Uganda National Council for Science and Technology (UNSCT # 2029) and Walter Reed Army Institute of Research (WRAIR #2327). Informed consent was obtained from all adult patients while a parent or legal guardian provided consent for patients below 18 years of age. Accompanying patient data for the samples were anonymized by removing all personal identifiers.
Demographic characteristics of enrolled volunteers
A total of 460 samples were selected from 1,485 archived serum samples by stratified random sampling, with each district forming strata were analyzed. Only serum with complementary data were selected. The demographic characteristics of the study participants are summarized in Table 1. The majority (54.8%) of the participants were children below five years, and the median age of the patients was 4.95 (95% CI 4-5%, age range 0.5 to 80 years). Majority (67.6%) of the patients came from residences classified as rural according to their reported villages, and there was balance in terms of gender. Half of the participants in this study were at preschool age, and another 30% were school children, making a total of 80% participants as minors (dependents).
Q-fever, SFG, TG and STG seroprevalence among the samples
The seroprevalence of the samples for Q-fever, SFG, TG and STG are presented in Table 2. Of the 460 serum samples analyzed for Q-fever, 35/460 (7.6%) were seropositive. Bwera hospital had highest seroprevalence (12.5%) whereas Mulago hospital had the lowest (2%). However, these observed differences between the sentinel sites were not statistically significant (Fisher´s exact = 0.075). For SFG, 29/460 (6.3%) of the serum samples tested were positive. Gulu and Bwera hospitals registered higher seroprevalences than the other hospitals. However, these observed differences were also not statistically significant (Fisher´s exact = 0.489). One serum sample of a 10-month-old male from Bwera hospital was reactive to both SFG and Q-fever antibodies. TG and STG had overall seroprevalence of 0.9% each (Table 2). Due to the low positive rates, the association between sites and serostatus were not assessed.
The sex, age and residence of the participants was assessed to establish possible association with positive outcome for SFG and Q-fever by bivariate logistic regression. Occupation was not assessed as 80% of the participants were infants or school children. Univariate analysis indicates that only location and partly age had higher odds of a patient having either Q-fever while age had increased risk for seropositivity to SFG (Table 3). The odds of seropositivity generally increased with age. Gender was not associated with serostatus for both Q-fever and SFG. Assessed together, only age (OR-adjusted=1.4, 95%CI=1.0-1.9, p=0.026) was significantly associated with Q-fever serostatus but not sex (OR-adjusted= 1.6, 95% CI= 0.8-3.4, p=0.181) and residence (OR-adjusted=0.4, 95% CI= 0.2-1.1, p=0.077). Again, higher odds for SFG serostatus with statistical significance were observed with age (OR-adjusted= 1.9, 95% CI= 1.4-2.6, p<0.001) but not sex (OR-adjusted= 1.5, 95% CI= 0.7-3.2, p= 0.338) and residence (OR-adjusted= 1.1, 95% CI= 0.5-2.6, p= 0.808). Associations between serostatus for TG and STG with patient factors were not assessed by logistic regression due to low positive rates.
Antibody titres
One serum sample from Gulu district had endpoint titre for Orientia IgM at 1:512, one other from Gulu and Bwera had 1:128 and the one from Bombo had 1:64.
The aim of this study was to ascertain the serostatus against Q-fever, rickettsioses and scrub typhus in febrile patients from five regional referral hospitals during the acute febrile illness surveillance period. This study is the first to demonstrate evidence of exposure of the Ugandan human population to Q-fever (7.6%), SFG rickettsia (6.3%), and STG (0.9%) of the 460 samples tested. We also found 0.9% exposure rate to TG rickettsia, corroborating the only other study to detect TG rickettsia in Mengo, Uganda in the 1950s [23]. The diseases caused by Rickettsia and Orientia are flu-like in manifestation and can range from mild to life-threatening yet remain difficult to diagnose [12]. These diseases are presumed to have a worldwide distribution and are likely to be endemic in sub-Saharan Africa, including Uganda. This serosurveillance study generates important baseline information on the occurrence of these diseases in Uganda, with the potential of being endemic, given the detection of rickettsial agents in ticks and fleas in Uganda [17,27,29,31]. This is vital to provide differentials to febrile illness and stimulate further research on the epidemiology of rickettsia groups that can then inform policy and best practices for clinical detection and management.
The sentinel sites used in this study are public regional referral hospitals that offered free primary healthcare to the population at the time of sampling [39]. Free care could have influenced the relatively high number of rural residents constituting the majority of febrile patients in this study, in contrast to the urban centres where private health facilities offer competitive or quicker healthcare services. Furthermore, the febrile patients were mainly children (54.8%) of 5 years and below and when expanded to include children up to 12 years, the proportion of febrile patients was 72.8%. This skewed age distribution of the patients could be a general reflection of Ugandan population structure in which 55 % of the population are children below 18 years [38] or it could be due to poor health seeking behavior of adults influenced by other factors such as out-of-pocket medical expenses. Given the lower age range of the study population and the number of seropositive detections across all rickettsia groups, these findings suggest recent ongoing infection.
The overall seroprevalence of Q-fever (7.6%) in this study was close to that in northern Tanzania (5%) [13], Kenya (8.9%) [9] and 6.9% in Bhutan [40]. The seroprevalence was significantly higher in Bwera hospital than in other hospitals possibly due to land use patterns and principal economic activities of the community served. Bwera hospital serves a population of both pastoralists and crop farmers, which may skew risk of exposure. The community living in the area is surrounded by queen Elizabeth National Park and the pastoralists often graze animals in the wildlife interfaces. Maina and others (2016) reported a higher prevalence of Q-fever in febrile patients from hospitals in pastoral communities in Kenya [9]. In this study, Q-fever exposure increased with age. This observation was similar to previous studies in Tanzania and Bhutan [40]. Although the seropositivity to Coxiella burnetii Phase 2 IgG may not constitute a clinical disease in an individual, these data suggest primary exposure of these individuals to the agent at some point. The patients could have been exposed through inhalation of the contaminated dust in animal yards where the agent which is stable in the environment secretions or ingestion of animal products such as milk and meat [34].
The overall SFG seroprevalence in this study was 6.3%. This finding is similar to the study in Tanzania [13] in which 8% of the febrile patients had SFG infection and 10% of the patients in Kenya were exposed [20]. However, the seroprevalence in this study was less than the 22.4% reported in children in Kenya [9]. Gulu hospital in the north and Bwera in the south-west had higher sentinel-based seropositivities than the rest. The predominant activities in these areas are farming and pastoralism. Similar to Q-fever, these findings could relate to land use, animal rearing activities and occupations that result in increased exposure to infected tick bites [18,19]. Advancing age was also associated with seropositivity to SFG in this study, similar to that observed in Kenya and elsewhere [41].
Our study found 0.9% positivity rate to Orientia infection by IFA. IFA is considered the reference standard test [42]. Although this is the first detection of STG positive case in Uganda, positive cases with relatively higher prevalence between 3.9-5% have been reported in the neighboring Kenya [9,20]. The positive rates in our study were too low to allow risk factor analysis. Additional research is required to better understand the circulating species of Orientia within this region. This study had some limitations. First, the serum samples used were collected at one-off encounter with the patient (acute-phase sample only). Convalescent serum would have better defined the cases. Secondly, due to cross-reactivity among different SFG rickettsia, it was not possible to identify specific pathogens.
We demonstrate that human populations in parts of Uganda are exposed to Q-fever and SFG and are likely to be endemic. TG and STG are present at low exposure levels. Greater awareness among clinicians about the occurrence of these diseases as potential differentials of undifferentiated febrile illness would improve patient management. Further studies on demonstrating Q-fever and SFG as the cause of fevers is required in addition to identifying the risk factors to inform prevention strategies.
What is known about this topic
- Rickettsioses are emerging infectious diseases transmitted by arthropods such as ticks, fleas, mites and lice - they are Gram-negative intracellular bacteria that cause febrile illnesses that range from mild to fatal or may complicate comorbidities in humans;
- Rickettsioses are difficult to diagnose. The infectious agents have been detected in arthropod vectors in parts of East Africa and the diseases in people have been reported in Kenya and Tanzania;
- In 2016, a case of a Slovenian traveller who visit Uganda was infected by African tick-bite fever and yet such cases have not reported within Ugandan population.
What this study adds
- The first evidence of exposure of Ugandan population to Q-fever (primarily a zoonotic agent acquired from discharges from animals), spotted fever and scrub typhus group rickettsia;
- This is the second time of reporting the exposure to endemic typhus in the country since the colonial times in 1950s;
- The overall seroprevalences human febrile patients was 7.6% to Q-fever, 6.3% SFG rickettsia, and 0.9% STG.
The authors declare no competing interests.
Denis Karuhize Byarugaba, Jeffrey William Koehler, Hannah Kibuuka, Edison Mworozi, Robert Tweyongyere and Wilfred Eneku: conceived and designed the study. Wilfred Eneku, Bernard Erima, Anatoli Maranda Byaruhanga, Gladys Atim, Titus Tugume, Qouilazoni Aquino Ukuli, Nora Gillian Cleary, performed the experiments. Wilfred Eneku, Nora Gillian Cleary, Michael Emery von Fricken, Denis Karuhize Byarugaba, analyzed the data. All the authors contributed to this work and have read and agreed to th efinal manuscript.
We thank the Staff of Makerere University Walter Reed Project working at the Emerging Infectious Diseases Laboratory and the sentinel Referral hospitals for the sample collection and processing. We acknowledge funding from US Department of Defense Threat Reduction Agency (Grant: HDTRA1-15-1-0043) to DKB under the "Acute Febrile Studies in Uganda", with additional support from the Armed Forces Health Surveillance Division (AFHSD) Global Emerging Infections Surveillance (GEIS) Branch under PROMIS ID P0038_22_KY to DKB.
Table 1: demographic characteristics of the study participants
Table 2: seropositivity of the samples for Q-fever, SFG, TG and STG by hospital. Table legend: aOne individual reacted to both Q-fever and SFG antibody ELISA tests and were considered as independent positives as cross-reactivity between the tests were not expected
Table 3: associations between patient demographic characteristics and Q-fever and SFG serostatus
Figure 1: map of Uganda showing the surveillance hospitals
- Parola P, Raoult D. Ticks and Tickborne Bacterial Diseases in Humans: An Emerging Infectious Threat. Clin Infect Dis. 2001 Mar 15;32(6):897-928. PubMed | Google Scholar
- Fang R, Blanton LS, Walker DH. Rickettsiae as Emerging Infectious Agents. Clin Lab Med. 2017 Jun;37(2):383-400. PubMed | Google Scholar
- Parola P, Raoult D. Tropical rickettsioses. Clin Dermatol. 2006 May-Jun;24(3):191-200. PubMed
- Gillespie JJ, Beier MS, Rahman MS, Ammerman NC, Shallom JM, Purkayastha A, et al. Plasmids and rickettsial evolution: insight from Rickettsia felis. PLoS One. 2007 Mar 7;2(3):e266. PubMed | Google Scholar
- Brown LD, Macaluso KR. Rickettsia felis, an Emerging Flea-Borne Rickettsiosis. Curr Trop Med Rep. 2016;3:27-39. PubMed
- Tomassone L, Portillo A, Nováková M, De Sousa R, Oteo JA. Neglected aspects of tick-borne rickettsioses. Parasit Vectors. 2018 Apr 24;11(1):263. PubMed | Google Scholar
- Parola P, Paddock CD, Socolovschi C, Labruna MB, Mediannikov O, Kernif T, et al. Update on tick-borne rickettsioses around the world: a geographic approach. Clin Microbiol Rev. 2013 Oct;26(4):657-702. PubMed | Google Scholar
- Abdad MY, Abdallah RA, Karkouri KE, Beye M, Stenos J, Owen H, et al. Rickettsia gravesii sp. nov.: a novel spotted fever group rickettsia in Western Australian Amblyomma triguttatum triguttatum ticks. Int J Syst Evol Microbiol. 2017 Sep;67(9):3156-3161. PubMed | Google Scholar
- Maina AN, Farris CM, Odhiambo A, Jiang J, Laktabai J, Armstrong J, et al. Q Fever, Scrub Typhus, and Rickettsial Diseases in Children, Kenya, 2011-2012. Emerg Infect Dis. 2016 May;22(5):883-6. PubMed | Google Scholar
- Robinson MT, Satjanadumrong J, Hughes T, Stenos J, Blacksell SD. Diagnosis of spotted fever group Rickettsia infections: the Asian perspective. Epidemiol Infect. 2019;147:e286. PubMed | Google Scholar
- Paris DH, Dumler JS. State of the art of diagnosis of rickettsial diseases: the use of blood specimens for diagnosis of scrub typhus, spotted fever group rickettsiosis, and murine typhus. Curr Opin Infect Dis. 2016 Oct;29(5):433-9. PubMed | Google Scholar
- Luce-Fedrow A, Mullins K, Kostik AP, St John HK, Jiang J, Richards AL. Strategies for detecting rickettsiae and diagnosing rickettsial diseases. Future Microbiol. 2015 Apr;10(4):537-64. PubMed | Google Scholar
- Prabhu M, Nicholson WL, Roche AJ, Kersh GJ, Fitzpatrick KA, Oliver LD, et al. Q fever, spotted fever group, and typhus group rickettsioses among hospitalized febrile patients in northern Tanzania. Clin Infect Dis. 2011 Aug;53(4):e8-15. PubMed | Google Scholar
- Biggs HM, Behravesh CB, Bradley KK, Dahlgren FS, Drexler NA, Dumler JS, et al. Diagnosis and Management of Tickborne Rickettsial Diseases: Rocky Mountain Spotted Fever and Other Spotted Fever Group Rickettsioses, Ehrlichioses, and Anaplasmosis - United States. MMWR Recomm Rep. 2016 May 13;65(2):1-44. PubMed | Google Scholar
- Rauch J, Eisermann P, Noack B, Mehlhoop U, Muntau B, Schäfer J, et al. Typhus Group Rickettsiosis, Germany, 2010-2017¹. Emerg Infect Dis. 2018;24(7):1213-20. PubMed | Google Scholar
- Walker AR, Bouattour A, Camicas JL, Estrada-peña A, Horak IG, Latif A, et al. Ticks of domestic animals in Africa: a guide to identification of species. The University of Edinburgh. Bioscience Reports, Edinburgh Scotland,U.K. 2003. 227 p. Google Scholar
- Eneku W, Erima B, Byaruhanga AM, Atim G, Tugume T, Ukuli QA, et al. Wide distribution of Mediterranean and African spotted fever agents and the first identification of Israeli spotted fever agent in ticks in Uganda. PLoS Negl Trop Dis. 2023 Jul 27;17(7):e0011273. PubMed | Google Scholar
- Horton KC, Jiang J, Maina A, Dueger E, Zayed A, Ahmed AA, et al. Evidence of Rickettsia and Orientia Infections Among Abattoir Workers in Djibouti. Am J Trop Med Hyg. 2016 Aug 3;95(2):462-5. PubMed | Google Scholar
- Teoh YT, Hii SF, Stevenson MA, Graves S, Rees R, Stenos J, et al. Serological evidence of exposure to Rickettsia felis and Rickettsia typhi in Australian veterinarians. Parasit Vectors. 2017 Mar 13;10(1):129. PubMed | Google Scholar
- Thiga JW, Mutai BK, Eyako WK, Ng'Ang'A Z, Jiang J, Richards AL, et al. High seroprevalence of antibodies against spotted fever and scrub typhus bacteria in patients with febrile Illness, Kenya. Emerg Infect Dis. 2015 Apr;21(4):688-91. PubMed | Google Scholar
- Heinrich N, Dill T, Dobler G, Clowes P, Kroidl I, Starke M, et al. High seroprevalence for spotted fever group rickettsiae, is associated with higher temperatures and rural environment in Mbeya region, Southwestern Tanzania. PLoS Negl Trop Dis. 2015 Apr 7;9(4):e0003626. PubMed | Google Scholar
- Masakhwe C, Linsuwanon P, Kimita G, Mutai B, Leepitakrat S, Yalwala S, et al. Identification and Characterization of Orientia chuto in Trombiculid Chigger Mites Collected from Wild Rodents in Kenya. J Clin Microbiol. 2018 Nov 27;56(12):e01124-18. PubMed | Google Scholar
- BAIRD RB, TONKIN IM. Endemic typhus in Mengo District, Uganda. East Afr Med J. 1951 Apr;28(4):157-67. PubMed | Google Scholar
- Sezi CL, Nnochiri E, Nsanzumuhire E, Buttner D. A small outbreak of louse typhus in Masaka District, Uganda. Trans R Soc Trop Med Hyg. 1972;66(5):783-8. PubMed | Google Scholar
- Banegura FK, Munube GM, Senyakazana AG, Nzaro E. An epidemic of typhus in Ankole, Uganda, 1975-1976. East Afr Med J. 1983 Jan;60(1):55-9. PubMed
- Bogovic P, Lotric-Furlan S, Korva M, Avsic-Zupanc T. African Tick-Bite Fever in Traveler Returning to Slovenia from Uganda. Emerg Infect Dis. 2016 Oct;22(10):1848-9. PubMed | Google Scholar
- Nakao R, Qiu Y, Igarashi M, Magona JW, Zhou L, Ito K, Sugimoto C. High prevalence of spotted fever group rickettsiae in Amblyomma variegatum from Uganda and their identification using sizes of intergenic spacers. Ticks Tick Borne Dis. 2013 Dec;4(6):506-12. PubMed | Google Scholar
- Socolovschi C, Matsumoto K, Marie JL, Davoust B, Raoult D, Parola P. Identification of Rickettsiae, Uganda and Djibouti. Emerg Infect Dis. 2007 Oct;13(10):1508-10. PubMed | Google Scholar
- Proboste T, Kalema-Zikusoka G, Altet L, Solano-Gallego L, Fernández De Mera IG, Chirife AD, et al. Infection and exposure to vector-borne pathogens in rural dogs and their ticks, Uganda. Parasit Vectors. 2015 Jun 5;8:306. PubMed | Google Scholar
- Corrigan J, Marion B, English J, Eneku W, Weng JL, Rugg M, et al. Minimal Rickettsial Infection Rates and Distribution of Ticks in Uganda: An Assessment of the Seasonal Effects and Relevance to Tick-Borne Disease Risk in East Africa. J Med Entomol. 2023 Jan 12;60(1):185-192. PubMed | Google Scholar
- Palomar AM, Cevidanes A, Portillo A, Kalema-Zikusoka G, Chirife AD, Romero L, et al. High Prevalence of Rickettsia spp. in Dog Fleas (Siphonaptera: Pulicidae) in Rural Uganda. J Med Entomol. 2017 Jul 1;54(4):1076-1079. PubMed | Google Scholar
- Anderson A, Bijlmer H, Fournier PE, Graves S, Hartzell J, Kersh GJ, et al. Diagnosis and management of Q fever--United States, 2013: recommendations from CDC and the Q Fever Working Group. MMWR Recomm Rep. 2013 Mar 29;62(RR-03):1-30. Erratum in: MMWR Recomm Rep. 2013 Sep 6;62(35):730. PubMed | Google Scholar
- Deyell MW, Chiu B, Ross DB, Alvarez N. Q fever endocarditis: a case report and review of the literature. Can J Cardiol. 2006 Jul;22(9):781-5. PubMed | Google Scholar
- Bwatota SF, Elizabeth AJC, Bronsvoort BM de C, Wheelhouse N, Luis E Hernandez-Castor and GMS. Epidemiology of Q-fever in domestic ruminants and humans in Africa. A systematic review. CABI One Health. 2022;(August):1-17. Google Scholar
- Duron O, Sidi-Boumedine K, Rousset E, Moutailler S, Jourdain E. The Importance of Ticks in Q Fever Transmission: What Has (and Has Not) Been Demonstrated? Trends Parasitol. 2015 Nov;31(11):536-552. PubMed | Google Scholar
- Ministry of Health. Uganda Clinical Guidelines 2016. Retrieved on 21/6/2018. Ministry of Health, Uganda. 2016. Accessed 19th August 2023.
- Thrusfield M. Veterinary Epidemiology. Butterworth-Heinemann Ltd., Elsevier Ltd. 1986;20-29.
- Uganda Bureau of Statistics. National Population and Housing Census 2014. Uganda Bureau of Statistics, Main Report, Kampala, Uganda. 2016. Accessed 19th August 2023.
- Institute for Health Metrics and Evaluation (IHME). Health Service Provision in Uganda: Assessing Facility Capacity, Costs of Care, and Patient Perspectives. Seatle, WA 98121; 2014.
- Tshokey T, Stenos J, Durrheim DN, Eastwood K, Nguyen C, Graves SR. Seroprevalence of rickettsial infections and Q fever in Bhutan. PLoS Negl Trop Dis. 2017 Nov 27;11(11):e0006107. PubMed | Google Scholar
- Tshokey T, Stenos J, Tenzin T, Drukpa K, Gurung RB, Graves SR. Serological Evidence of Rickettsia, Orientia, and Coxiella in Domestic Animals from Bhutan: Preliminary Findings. Vector Borne Zoonotic Dis. 2019 Feb;19(2):95-101. PubMed | Google Scholar
- Koh GC, Maude RJ, Paris DH, Newton PN, Blacksell SD. Diagnosis of scrub typhus. Am J Trop Med Hyg. 2010 Mar;82(3):368-70. PubMed | Google Scholar
Search
This article authors
On Pubmed
- Wilfred Eneku
- Bernard Erima
- Anatoli Maranda Byaruhanga
- Nora Gillian Cleary
- Gladys Atim
- Titus Tugume
- Qouilazoni Aquino Ukuli
- Hannah Kibuuka
- Edison Mworozi
- Christina Douglas
- Jeffrey William Koehler
- Michael Emery von Fricken
- Savino Biryomumaisho
- Robert Tweyongyere
- Fred Wabwire-Mangen
- Denis Karuhize Byarugaba
On Google Scholar
- Wilfred Eneku
- Bernard Erima
- Anatoli Maranda Byaruhanga
- Nora Gillian Cleary
- Gladys Atim
- Titus Tugume
- Qouilazoni Aquino Ukuli
- Hannah Kibuuka
- Edison Mworozi
- Christina Douglas
- Jeffrey William Koehler
- Michael Emery von Fricken
- Savino Biryomumaisho
- Robert Tweyongyere
- Fred Wabwire-Mangen
- Denis Karuhize Byarugaba